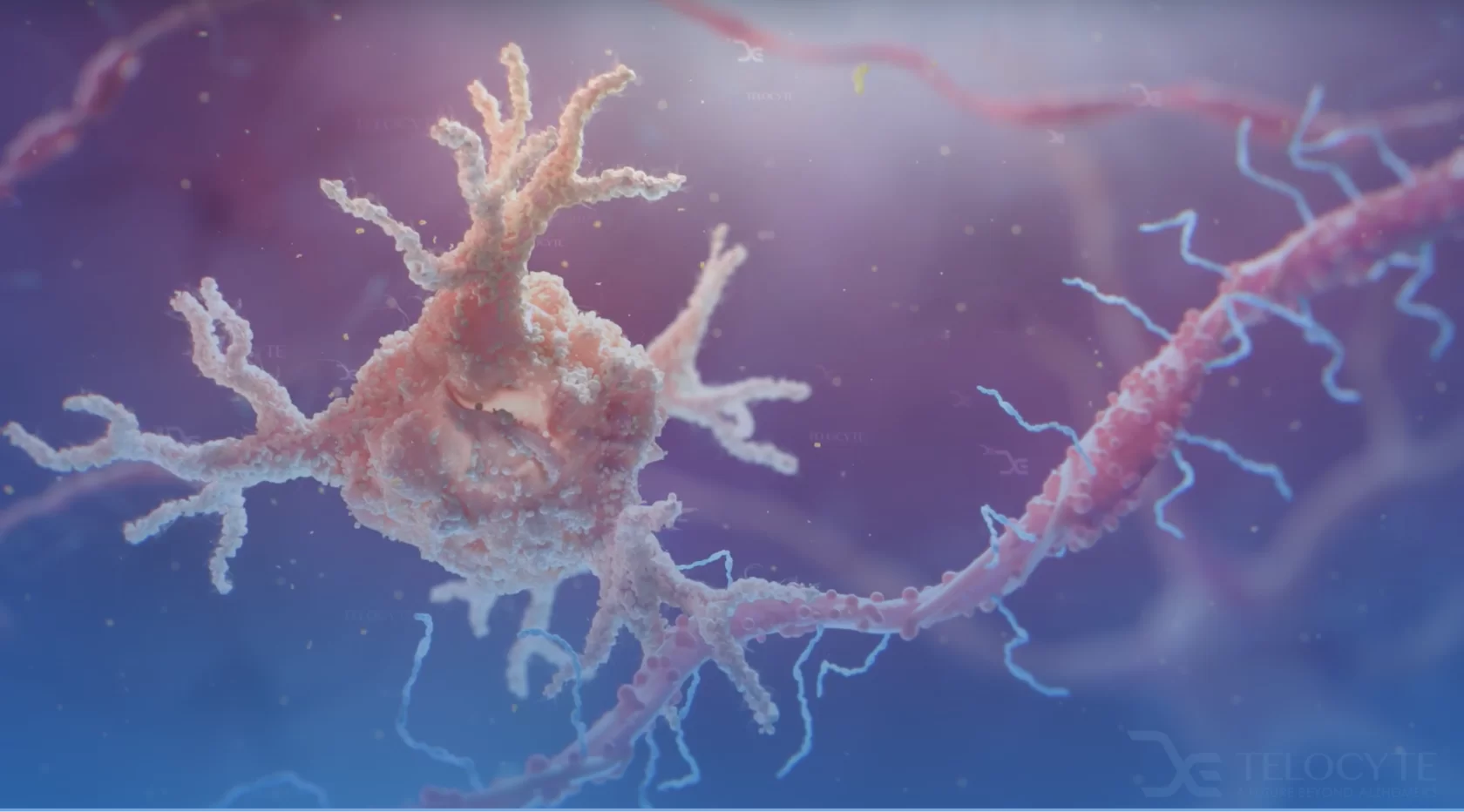
The Science
ultimately caused by cell aging. In the case of
Alzheimer's disease, glial cells age over time
and trigger a cascade of pathology.
How Alzheimer's works
"A Unified Model Of Dementias And Age-Related Neurodegeneration" was published in Alzheimer's & Dementia in 2020, providing systematic explanation of how Alzheimer's works, a model that is consistent with all known clinical data, and which has accurately predicted the outcome of other trials, such as those focused on amyloid, tau proteins, and other clinical targets.
Central to the model is that cell aging drives the cascade of cellular pathology. Aging itself, as well as a host of other factors, push cells – such as microglia – to divide. As cells divide, their telomeres shorten, triggering gradual, but pervasive changes in gene expression. The result is a slowing of molecular turnover, permitting an increase in molecular damage, increasingly dysfunctional cells, and secondary problems in neurons that rely upon normal glial cell function. Glia become increasingly dysfunctional and unable to deal with Beta-amyloid, tau proteins, and other molecules that require clearance.
As a result of the accumulation of amyloid plaque the growth of tau tangles, and a host of other problems that result from cell aging, there is a gradual loss of the synaptic networks and neurons that underlies the age-related dementias, including Alzheimer's. Alzheimer's has been, until now, not only a personal tragedy, but a clinical enigma. Once we understand the disease, however, effective intervention can follow.
Using telomerase therapy, we can reset gene expression, allowing cells to clear the proteins that define the pathology, and reversing the pathology before it can progress. Human trials will administer a telomerase gene, which will relengthen telomeres within glia and neurons and reset gene expression. Never before has any therapy offered the potential to not only prevent, but potentially reverse Alzheimer's disease. Animal trials show that we can not only prevent, but reverse at least some of the CNS dysfunction.
Age itself is the greatest single risk factor for age-related disease, but the fundamental aging process is often ignored. The question of how age results in the diseases of aging Is more complicated than we realize.
Our genes - our specific alleles - can increase or decrease our risk of age-related disease, but they do so in the way they play out in the context of cellular aging. Some alleles (such as APOE4) can increase the rate of cell damage as the cells of our nervous system age, while other alleles (such as APOE2) result in a comparatively lower rate of cell damage even at equivalent chronologic ages.
Environment toxins, like chemotherapy (toxins aimed at cancer cells) can also damage normal cells, which accelerates cell division and the rate of cell aging. Hepatic toxins, for example, work by damaging liver cells; remaining liver cells thus divide to replace missing cells, increasing the rate of cell aging. A typical clinical example is the hepatic damage that can occur as a result of an acetaminophen overdose.
Hyperglycemia can directly damage cells, but is also indicative of a failure of cells to take in glucose appropriately. Direct damage to vascular, renal, and other cells results in cell failure and cell loss, prompting cell division and accelerating cell aging and increased cell dysfunction. The outcomes are myriad: renal failure, vascular disease, and age-related disease in multiple tissues.
Stress is a term that is often used loosely, yet any physiologic stress has a propensity to result in cell damage or cell loss in affected tissues. One example might be the immune system, where stress (even psychological stress) can increase the rate of turnover of circulating lymphocytes, thus accelerating cell aging and age-related problems.
We know far less about the microbiome and its implications for cell aging - than we should, but there are already indications that an abnormal microbiome can increase the rate of cell damage to some tissues and organs, accelerating cell division and cell aging, with clinical consequences.
Trauma damages and often results in the death of cells. This can occur, for example in the case of trauma to the skin, the joints, the brain, etc. Especially is this trauma is repetitive or continual, the result is cell division to replace lost cells, thus accelerating cell aging and age-related disease. Examples are repetitive head injuries (and the risk of dementia),high-impact repetitive sport injuries to our joints (and the risk of osteoarthritis),as well as the rheologic injury to vascular endothelial cells in the case of hypertension (and the risk of age-related vascular disease, such a aneurysms, infarction, or stroke)
The most common example of radiation damage is that induced by ultraviolet exposure in our skin. Prolonged and/or repetitive ultraviolet damage (for example sunburn or simply years of exposure even without sunburn) induces early skin aging. Other forms of radiation, radiation therapy for example, are also known to accelerate cell aging.
Ironically, senolytic therapy may accelerate cell aging by removing those cells that are already aged. The laudable intent is remove cells that cause tissue dysfunction, but the unavoidable implication is that remaining cells must divide to replace the lost cells, thus accelerating cell aging of the remaining cell population. The initial outcome may offer clinical benefits, but the long-term outcome is likely to be accelerated pathology in the treated tissue.
Any hormonal abnormality (for example hypothyroidism or hyperthyroidism, adrenal abnormalities, pituitary abnormalities, etc.) increases the risk of cell dysfunction in affected tissues and, over time, accelerated cell loss with consequent cell replacement, accelerated cell aging, and age-related disease
Infectious agents, whether bacteria, virus, fungi, chlamydia, or prion, can not only accelerate immune system aging, but induce direct damage to affected tissues. Thus, a history of encephalitis or other CNS infection increases the risk of dementia. Anytime there is loss of cells due to infection, as can occur in any organ, the result is cell division, accelerated cell aging, and age-related disease.
Hypertension stresses the vascular endothelial cells that line our blood vessels. As these cells are lost due to rheologic injury, the remaining cells divide and replace the missing endothelial cells, thus accelerating cell aging. The outcome is that such cells become increasing dysfunctional and precipitate age-related vascular diseases: aneurysms, coronary artery disease, strokes, myocardial infarction, congestive heart failure, peripheral capillary pruning, etc.
Not only are there other variables that can accelerate cell aging, but many variables are interactive or, like hyperglycemia, are both dependent and independent variables, having multiple effects throughout the complex cascade of pathology.
variables
Cell senescence is a continuum from young, functional cells to old, dysfunctional cells. The enpoint of this continuum is replicative senescence(when the cell can no longer divide), but cell senescence is a gradual process, not simply the end of the process. As cells senesce, their telomeres gradually shorten, which alters the overall pattern of gene expression, slowing cellular maintenance mechanisms, and resulting in increasingly dysfunctional cells
Telomeres (the last several thousand base pairs at the ends of our chromosomes) shorten with each cell division, so cells show telomere shortening over time. This relative loss of telomere length drives the gradual but pervasive change in gene expression that define cell senescence. Cell senescence causes age-related disease.
The crucial difference between a young cell and an old cell is not the genes, but the gene expression. If a cell was a symphony orchestra, and each gene were an instrument in that orchestra, then the difference between a young cell and an old cell is not the instruments, but that score those instruments are playing. The telomere functions as the "conductor" of the genetic orchestra and both the score and the tempo of an old cell is different from that of a young cell. The difference between a young cell and an old cell is not genetic, but epigenetic and the result of that difference is a gradual loss of cell maintenance.
As cells age, they become dysfunctional, largely as a result of a gradual but pervasive failure of maintenance. The core of this failure is the gradual slowing of molecular turnover, as a result of epigenetic shifts. As telomeres shorten, gene expression changes (the epigenetic shift), molecular turnover slows, allowing damage to accumulate, and cell function to degrade. Cell dysfunction - caused by the slowing of molecular turnover results in tissue and organ changes which we see clinically as age-related disease.
INTERVENTION
Aging tissues demonstrate a plethora of inflammatory changes, in the setting of a general increase in measures of inflammation, but also including inappropriate onset and resolution of inflammation in response to stimuli. In some respects, such changes have come to define cell aging, although best seen as downstream biomarkers of the epigenetic changes that define cell aging, rather than a cause of cell aging
Not only does the aging cell produce more ROS and leak them more easily from the mitochondria, but the molecules responsible for scavenging and removing these ROS, such as superoxide dismutase and catalase, are themselves being turned over more slowly. As these molecules are replaced more slowly, the pool of scavenger molecules becomes less efficient, permitting an increase in the damage caused by ROS that aren't captured in time.
Advanced glycation end products are merely one of dozens of downstream "symptoms" of cell aging. These molecules, bound to sugars, are recycled more rapidly in young cells, but are less rapidly dealt with as a result of cell aging.
Mitochondrial dysfunction, resulting from the slower turnover rate of required enzymes produced in the nucleus, has critical consequences. There is a gradual increase in the percentage of ineffective mitochondrial enzymes, less efficient ATP production, and a gradual increase in the rate of free radical (ROS) production. For any given input of metabolic fuel - glucose for example - the ATP/ROS ratio show a gradual but inexorable fall as a direct result of cell aging and epigenetic shifts in the cell nucleus.
Mitochondria produce the majority of ROS. As lipid turnover slows, however, the mitochondrial membrane becomes increasingly porous, permitting ROS to escape into the cytoplasm in increasing numbers, an increasing problem for the entire cell.
Like other molecules, the lipid molecules that are the building blocks of cell membranes also show a slower rate of turnover as a result of the epigenetic changes that define cell aging. The result is a gradual increase in damaged molecules within such membranes, including the cell membrane, the mitochondrial membrane (permitting more ROS to escape into the cytoplasm), and the nuclear membrane (permitting more ROS to enter the nucleus, thus increasing the rate of DNA damage).
Lipofuscin might be thought of as the indigestible "garbage" leftover when cells insufficiently degrade some lipids. In some ways the problem is a parallel to what occurs in the case of beta amyloid: as cells age, their rate and efficiency of molecular turnover decreases, and the result is a gradual increase in such damaged molecules, which are visible within and between affected cells.
Beta amyloid plaques form due to two variables: the degree of cell aging (which slows the turnover of beta amyloid molecules) and the specific type of beta amyloid produced (APOE4 alleles result in an increased rate of aggregation). The more advanced the degree of cell aging and the "stickier" the molecules, the earlier the onset of plaques and clinical dementia. Such plaques are a symptom, rather than the cause of dementia.
Mitochondrial dysfunction is increasingly recognized as a prominent feature in age- related diseases. The majority of mitochondrial enzymes derive from the nucleus and, as with other molecules, show a decelerated turnover in cell aging. The result is a gradual increase in the percentage of dysfunctional enzymes, the outcome being a dysfunctional mitochondria, a dysfunctional cell, a dysfunctional tissue, and increasingly apparent clinical disease.
DNA damage occurs constantly and in all cells, but is offset by constant - and almost flawless - DNA repair. The rate of repair - effected by a panoply of DNA repair enzymes - decreases with age, however, resulting in a gradual but exponential increase in mutations and as a clinical outcome, cancer. The decrease in the rate of DNA repair is a direct result of cell aging, as the turnover rate of the key enzymes slows.
A classic histologic finding, seen in the substantia nigra in patients with Parkinson's, these aggregates are, like other findings, likely to be the result of cell aging. While they can serve as the defining pathology (along with loss of dopaminergic neurons) of Parkinson's they are an outcome rather than the underlying cause of cellular dysfunction and dementia.
Like beta amyloid aggregates, tau tangles increase as a result of cell aging. As cells age, the rate of molecular turnover slows, damage begins to accrue, and the result is increasing dysfunctional and damages neurons in the CNS. While these tangles have direct implications for neuronal function, they are themselves the downstream outcome of cell aging. In that respect, tau tangles are merely a key symptom, not the cause, of dementia.
The cells of the vascular endothelium are one of the earliest sites of measurable change in age-related vascular disease Such disease can be predicted by cell aging (as measured by telomere loss, for example), changes which predict and precede gross histologic pathology as well as clinical findings, such as aneurysm, atherosclerosis, etc.
There are hundreds of biomarkers that correlate with aging and, more specifically, result from cell aging. These biomarkers are not, however, causes of aging anymore than symptoms (e.g., a fever) is the cause of an infection (e.g., Covid 19 infection). The most effective point of intervention is to understand the upstream cause of age-related disease rather than the downstream biomarkers.
BIOMARKERS
Bibliography

The Telomerase
Revolution
Meant for the general public, this book describes what we know about aging — and how to prevent and reverse age-related diseases using telomerase therapy. It takes the reader "to the front lines of the upcoming revolution in medicine" and gives us "a detailed but highly accessible scientific journey, providing startling insights into the nature of human aging". The Telomerase Revolution is the complete work on the latest science on human aging, covering both the theory and the clinical implications.
Cells, Aging,
and Human Disease
PhD Oxford University Press, 2004
Cells, Aging, and Human Disease (Oxford University Press) is the first textbook to explore aging all the way from genes to clinical application, analyzing the fundamental cellular changes which underlie human age-related disease.
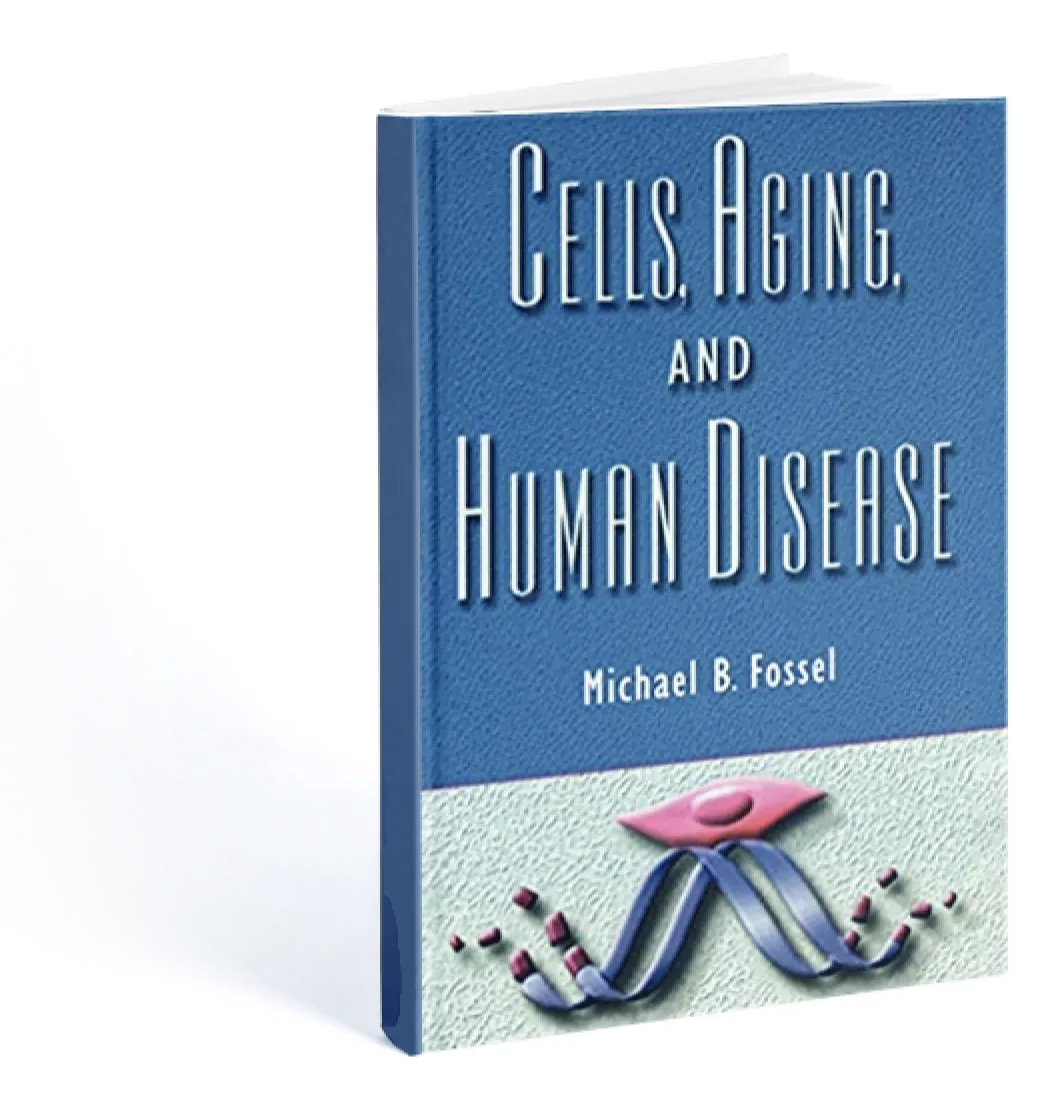
Scientific Articles
This article reviews the role of telomeres in age-related disease and discusses the potential of telomere-base clinical interventions.
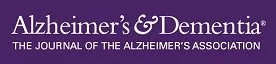
This article reviews the role of telomeres in age-related disease and discusses the potential of telomere-base clinical interventions.
This article reviews the role of telomeres in age-related disease and discusses the potential of telomere-base clinical interventions.
This article reviews the role of cell senescence in age-related disease and discusses the risk of senolytic-based therapies.
This article shows that telomerase activation using AAV9 vectors and telomerase gene is not necessarily associated with increased cancer.

A key article by our chief collaborator, showing that we can use TEL1 to improve behavioral decline and improve CNS function.
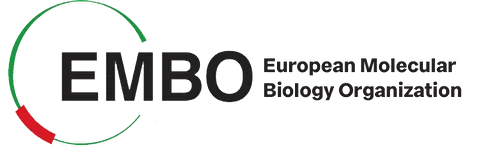
An article showing the ability of telomerase to reverse behavioral changes as well as cell and anatomic age-related changes in the CNS.
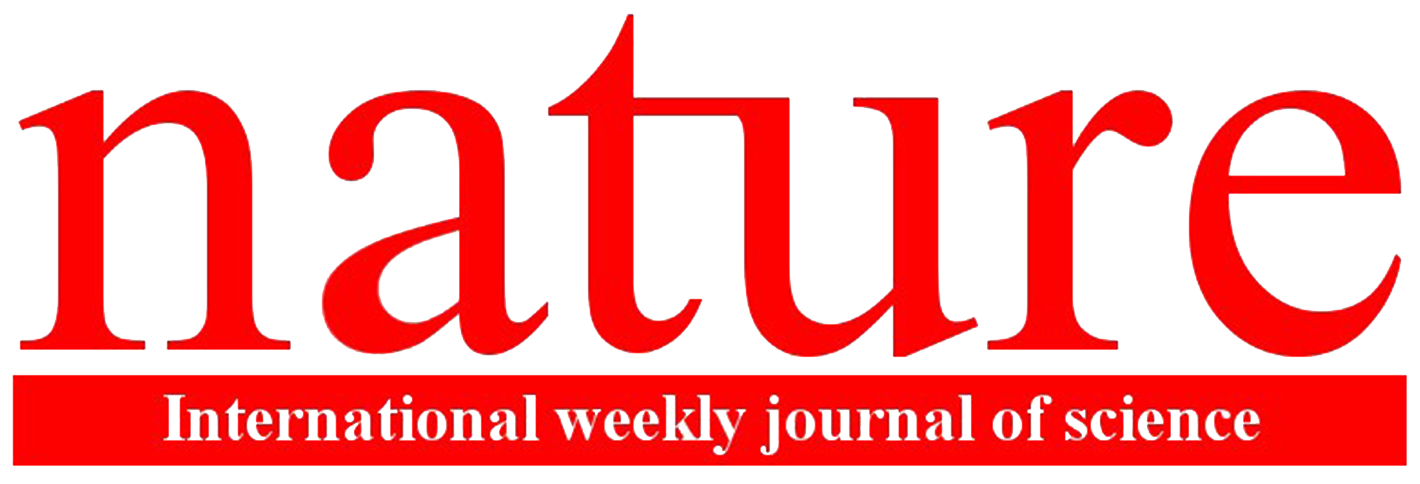
An article showing the ability of telomerase to reverse behavioral changes as well as cell and anatomic age-related changes in the CNS.
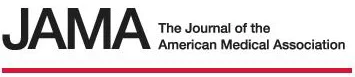
The first article detailing the techniques and propose their use in curing Alzheimer's and other age-related disease.
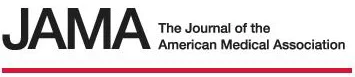
This article explains why telomere length can drive disease, yet the naïve use of telomeres as a biomarker can be difficult, if not actually misleading.

Effective clinical intervention requires an understanding of disease processes. This article reviews the requirements for a model and its clinical implications.
